POLICY BRIEF | MARCH 2022
Shipping decarbonisation shout for new technologies: developing passive air lubrication
EXECUTIVE SUMMARY
To achieve the greenhouse gas (GHG) emissions reduction goal of achieving net zero by 2050 as outlined in the European Green Deal, the maritime shipping industry must reduce emissions: 50% by 2030 and 90% by 2050. This cannot be achieved by a single measure alone.
- Ship resistance reduction measures (via advanced coatings, air lubrication, hull management of biofouling, hull form design) are necessary drivers available to reduce emissions today. Ship owners cannot alone focus on alternative fuels, which will not be available in the medium-term in large quantities, and slow steaming which is well understood but will increase delivery times and the number of ships needed for transport. Therefore, the need for resistance reduction measures is high now.
- The AIRCOAT project advances a bio-inspired green solution to create an air layer on ship hulls under water, so-called ‘air lubrication’, with the aim to reduce drag and to prevent biofouling, thus reducing fuel consumption and emissions.
- To realise market-ready innovative solution, longer research time-frames and incremental funding are necessary to allow for further improvements on real-world testing that is appropriate for the two-decade life cycle of ships.
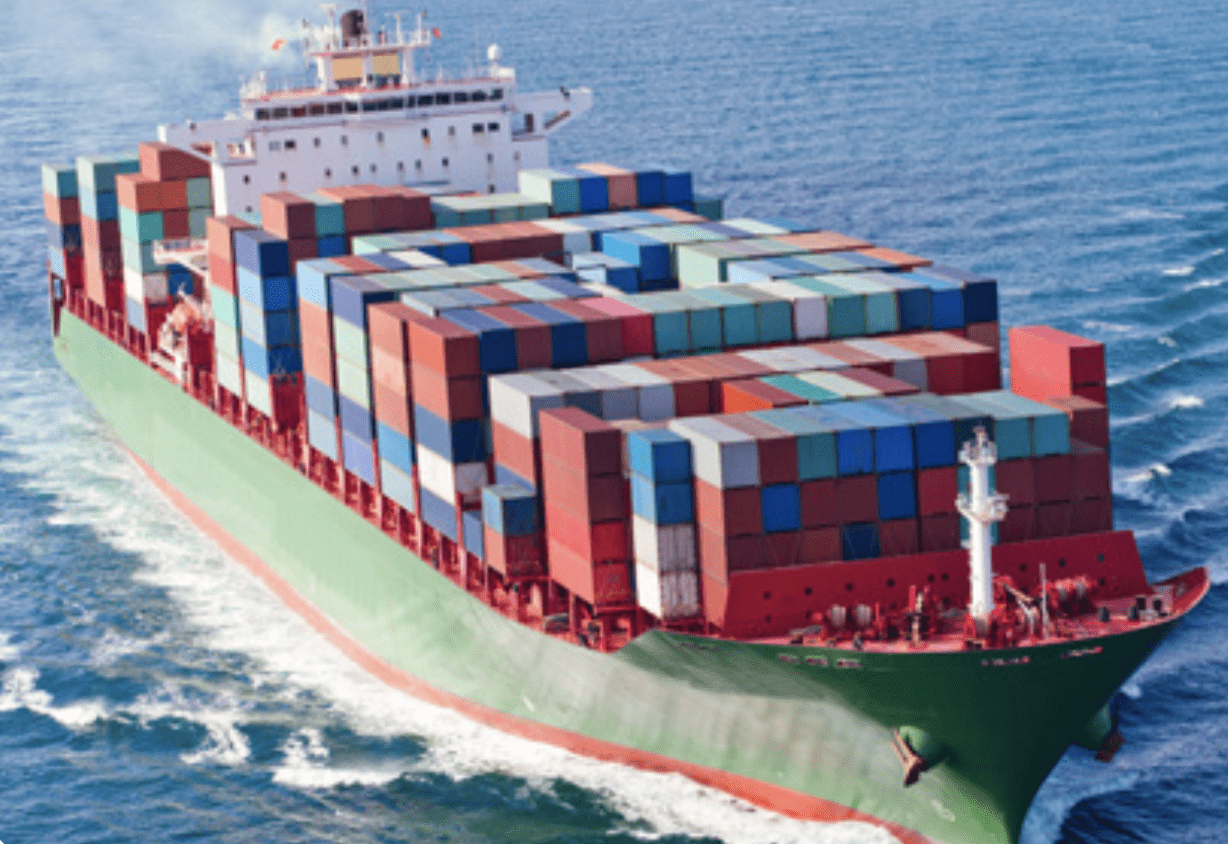
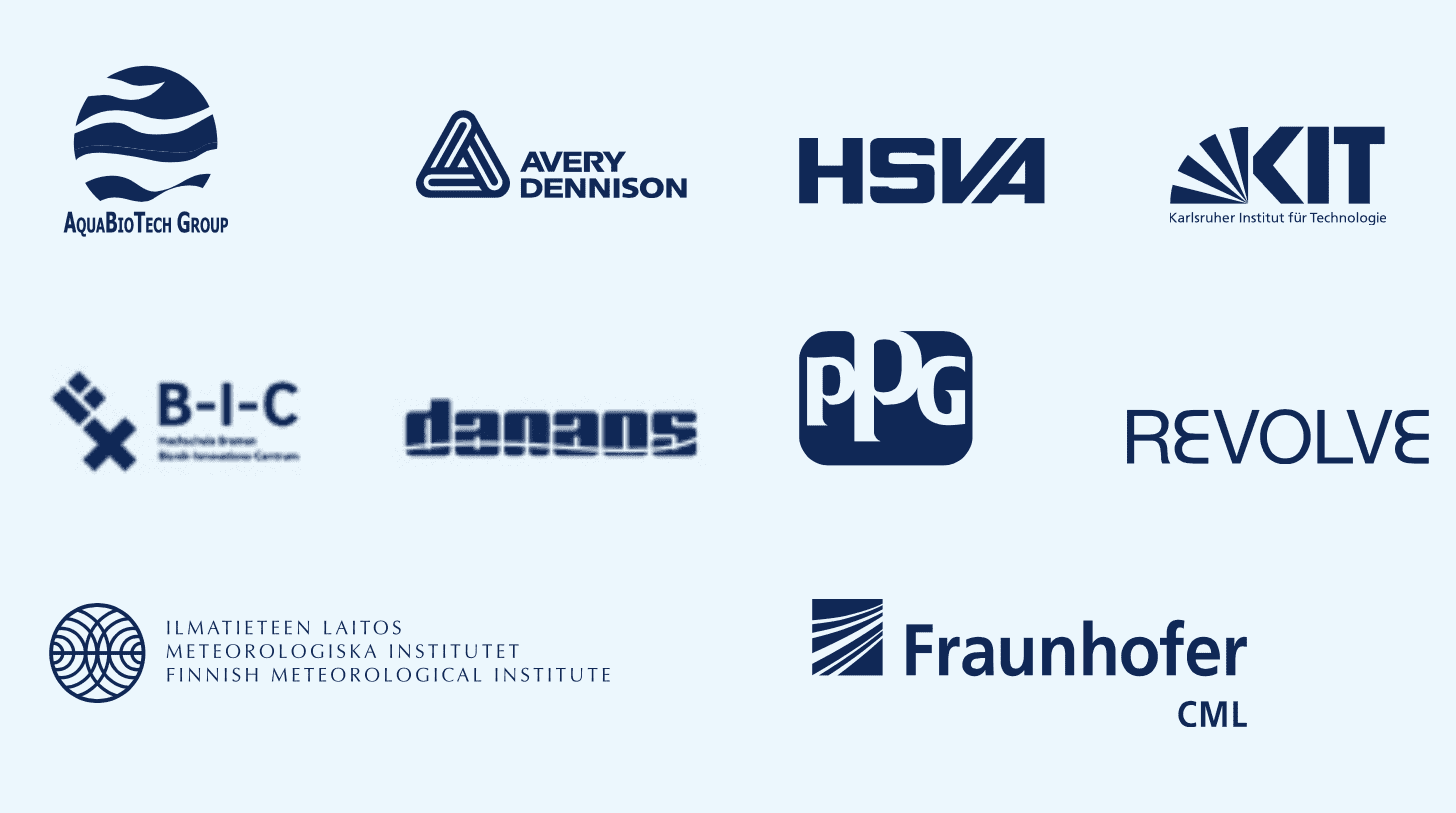
INTRODUCTION
In 2019, the European Green Deal was adopted to make Europe the first climate-neutral continent by reducing the EU’s greenhouse gas (GHG) emissions by at least 50% by 2030 and reaching zero net emissions by 2050, compared to 1990s levels. To achieve climate-neutrality, the transport sector needs to reduce emissions by 90% by 2050. However, so far transport has been one of the slowest sectors in terms of emissions reduction (transportenvironment.org), and the next decade will be critical in the transition to a low carbon economy (based on energy sources that produce low levels of GHG emissions). Therefore, the transport sector will need a combination of different technologies to achieve its goal. Therefore, the transport sector will need a combination of different technologies to achieve its goal.
A tempting option is saving energy by simply reducing the speed of the ships – the so-called slow steaming. There is no doubt that ships reducing their speed by a factor of two, for example, would need less energy for transport. But in this case, the time for delivery would increase by a factor of two as well and the number of ships needed would also be increased for partial compensation. Another interesting option is based on the use of alternative fuels. However, they will not come into play soon enough to reach 2030 goals needing too much time for implementation to be put into place within the 2020s to play its part in reaching EU climate goals (ec.europa.eu). Further options are Wind-Assisted Propulsion Systems, Electrification, Solar Power Technologies, Route optimisations and many others.
Advanced ship hull coatings are retrofit solutions available for the current fleet of ships to reduce their emissions and offer a viable opportunity to increase efficiency and reduce fuel consumption. As fouling may cause a 19% increase in fuel consumption each year per ship (after applying a new ship coating), focus on the use of anti-fouling solutions has been an important research focus (safety4sea). However, many of these solutions leach biocides into the water, and research into green solutions is critical to protect marine ecosystems from further several damage. To ensure the viability of these technologies in the real world, with 21-year ship life cycles and associated maintenance time and economic costs, it is crucial that research timeframes allow for appropriate testing to be carried out. These timeframes contradict with decarbonisation goals and funding calls often provide requirements that are difficult to reach (e.g. up to 35% GHG reduction compared to original ship design (ec.europa.eu)) with one measure alone, hence only the combination of promising technologies can be successful (e.g. CHEK H2020 project (projectchek)), however grant funding call budgets are limited and set the innovation potential low.
It is within this framework that the Horizon 2020 EU funded AIRCOAT project is developing a bio-inspired solution that aims to reduce friction on ships, lowering fuel consumption and thus GHG emissions. The AIRCOAT technology is based on a structured foil that retains air when submerged due to the lower viscosity (measure of resistance to the deformation of a fluid) of air in comparison to water, drag reduction is expected. Furthermore, the air layer acts as a physical barrier reducing attachment of maritime fouling organisms.
ECONOMIC AND POLICY CONTEXT
Maritime transport is the backbone of the global economy and accounts for the movement of 80% of all goods worldwide… and some 940 million tonnes of CO2 annually which is equal to approximately 2.5% of global GHG emissions. According to a GHG study by the International Maritime Organisation (IMO), world trade is predicted to grow by 9% between 2030 and 2050, with an estimated increase of maritime sector emissions between 90% and 130% by 2050 (imo.org).
The European shipping fleet, some 18,000 vessels, represents one fifth of the total world fleet in terms of dead weight tonnage (DWT) with 77% of European external trade being made by sea, making the continent one of the key parts of the international supply chain, and shipping a vital aspect of European trade (EEA 2021 (eea.europa.eu)). In terms of emissions, the 2021 European Maritime Transport Environmental Report (EMTER) found that ships calling at EU and European Economic Area ports generated approximately 18% of global maritime transport emissions in 2018 (EEA 2021).
Despite contributing less emissions than other modes of transport, it is vital that the shipping sector must work to reduce its GHG footprint to ensure that the expected growth for the sector in the coming decades does not hinder global and European carbon emissions mitigation efforts nor the competitiveness of the European economy (eea.europa.eu).
As part of its transition towards a carbon-neutral economy, Europe is dedicating funds to find environmentally-friendly solutions to reduce GHG emissions in maritime shipping. Under the Horizon 2020 and Horizon Europe programme, the length of the research funding is between 3-4 years. The ship-building sector is working on 5-to-6-year cycles to retrofit and 10-to-20-year cycles to build new ships. Therefore, adequate funding times are required to validate the research results and to run the proper experiments in laboratories and in real-world conditions.
GHG EMISSIONS REDUCTION STRATEGIES
To reduce GHG emissions, the shipping sector has several design, operational, and economic solutions to turn to increase fuel efficiency and reduce carbon intensity, such as Wind-Assisted Propulsion Systems, Electrification, Solar Power Technologies or Route optimisations. To meet emissions targets, the sector will need to employ a variety of these solutions at once and combined for optimal results, from alternative fuels and voyage optimisation, to fleet management and logistics incentives (un.org).
Among the array of options, very effective in terms of GHG emission reductions are alternative fuels, given the energy required for large ships travelling long distances. However, there are several barriers facing the uptake of fuel alternatives linked in large part to the diverse and international nature of the sector, which make the standardisation of technology and infrastructure difficult, slowing the uptake of novel solutions (waterborne.eu). Alternative fuels require expensive infrastructural changes and important long-term investments in different kinds of ship fitting, corresponding to different alternative fuels. In addition, international regulatory differences make the choice of alternative fuels to invest in difficult and unclear (advancefuel.eu). This also raises issues with differing priorities for various stakeholders focusing on different technologies (e.g. ship builders versus port managers).
The urgency to reduce emissions was made very clear in the recent Intergovernmental Panel on Climate Change (IPCC) report and as the 2030 deadline approaches, the shipping sector needs to look towards feasible options to meet the interim emissions goals. Moreover, with half of the EU fleet that is less than 15 years old, it is now imperative that operational and retrofit solutions are at the top of the priority list to avoid the early retirement of current ships during the transition period before the uptake of alternative fuels (eea.europa.eu).
A very effective strategy to improve fuel efficiency is hull resistance reduction measures, whereby the efficient use of fuel can be increased by reducing hull degradation and drag. Biofouling – the build-up of micro-organisms, algae, plants, or small marine animals – is one of the major causes of increased drag on ships. When biofouling occurs on a ship hull, there is an increase in roughness and thus drag. This can cause either a loss of ship speed or an increase in fuel needed to maintain ship speed, each incurring negative economic and environmental impacts through fuel consumption. Some estimates state that a loss of 10% of speed from biofouling can require as much as a 40% increase in fuel to counterbalance the effect and reach the original speed of the ship before fouling occurred (imo.org).
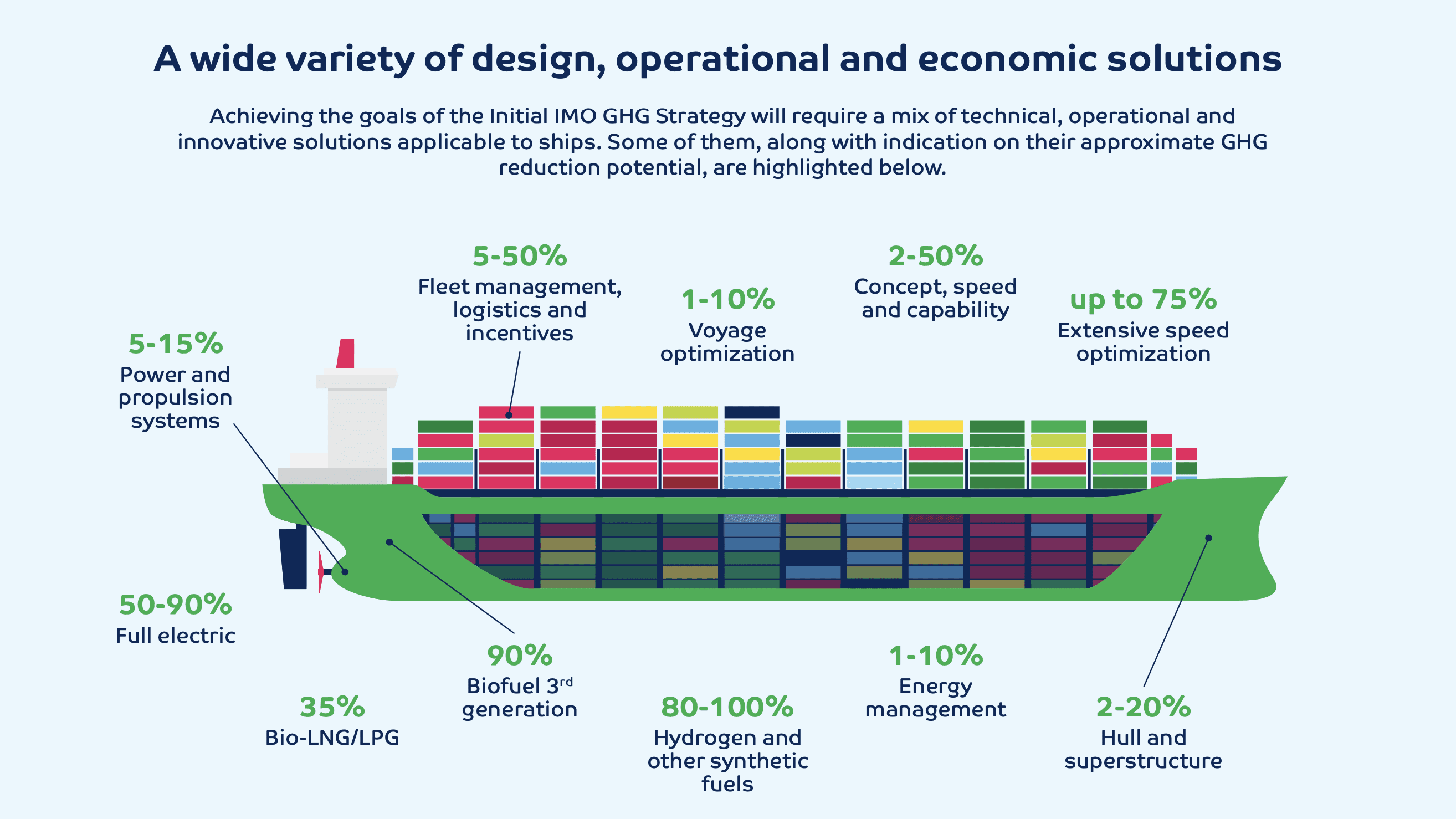
Source: Report on the preliminary results of the study on the Impact of Ships’ Biofouling on Greenhouse Gas Emissions, IMO, 2021.
According to the preliminary findings of a new study on the impact of biofouling on GHG emissions from the IMO launched at COP26, even a layer as thin as 0.5mm covering just 50% of the hull surface can causes an increase of 20-25% of GHG emissions, while more severe conditions, such as light layer of calcareous growth – e.g. barnacles or tubeworms – GHG emissions can increase up to 55%, depending on ship speed, characteristics and prevailing conditions (imo.org).
The extent of fouling depends on several factors like availability of sunlight, water temperature, salinity and fouling species. A clean steel surface will start to foul immediately upon exposure to sea water. Microbial film which forms first provides a basis for hard fouling which may include algae and marine invertebrates. These increase hull surface roughness and friction resistance, leading to abnormally large fuel consumption and air emissions.
Hull management includes the application of biofouling control strategies, including anti-fouling coatings and hull cleaning, and is an important aspect of fuel efficiency management. With regards to hull coatings, the industry has come a long way from the chemical anti-fouling paints that employed the use of toxic compounds that slowly leach into the seawater and kill barnacles and other sea life attached to the ship. The use of these persistent compounds were banned in the Antifouling Convention of the IMO, but they were replaced with other organometallic compounds (Cu, Zn) which are still toxic to marine organisms. Since then, industry has been looking towards more environmentally friendly – or in other words less toxic – options that do not compromise in efficacy.
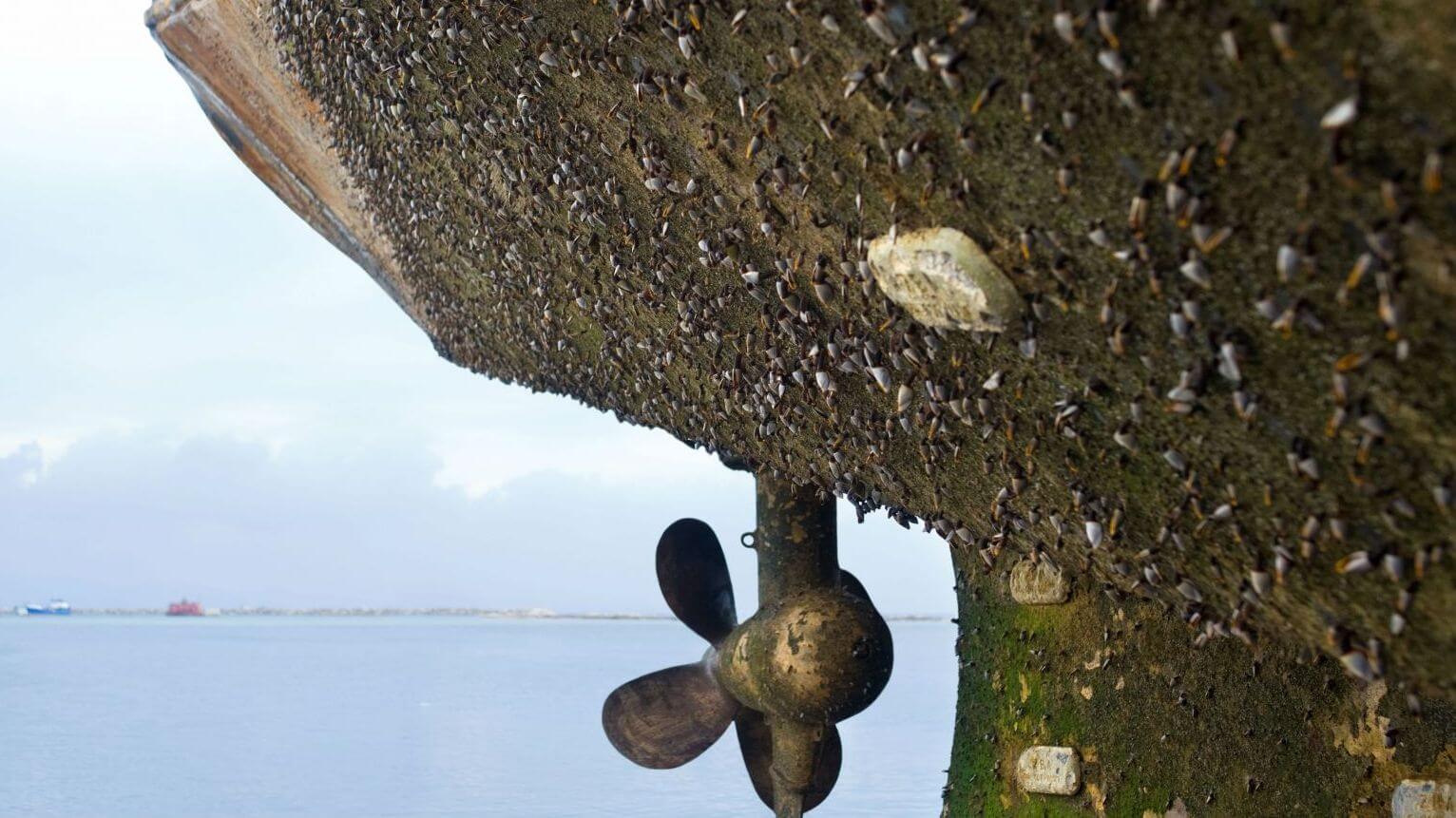
Biofouling on ship hull.Photo: Svetlana Yudina
AIRCOAT SCIENTIFIC RESEARCH APPROACH AND TECHNICAL RESULTS
The AIRCOAT project is developing an innovative technology for making ship hulls more fuel efficient and eco-friendly using a passive air lubrication. This technology coats the ship hull with a thin and permanent layer of air, which acts as an environmentally friendly lubricating layer. In this context, it is indented to realise a stable air layer under water, not needing continuous pumping or air bubbling.
The AIRCOAT technology is inspired by Salvinia Molesta – a floating water fern – that forms a permanent air layer when submerged in water. The air retaining ability of the Salvinia effect relies on a complex micro- and nano-structured surface with hydrophobic and hydrophilic characteristics. AIRCOAT implemented this natural phenomenon technically to produce a bio-inspired hull coating technology which combines three key advantages:
- Lubrication of the ship hull with a layer of air reducing friction.
- AIRCOAT is expected to reduce fouling, as fouling organisms tend to settle on a solid surface and noton a layer of air.
- This approach does not apply toxic substances to decrease fouling.
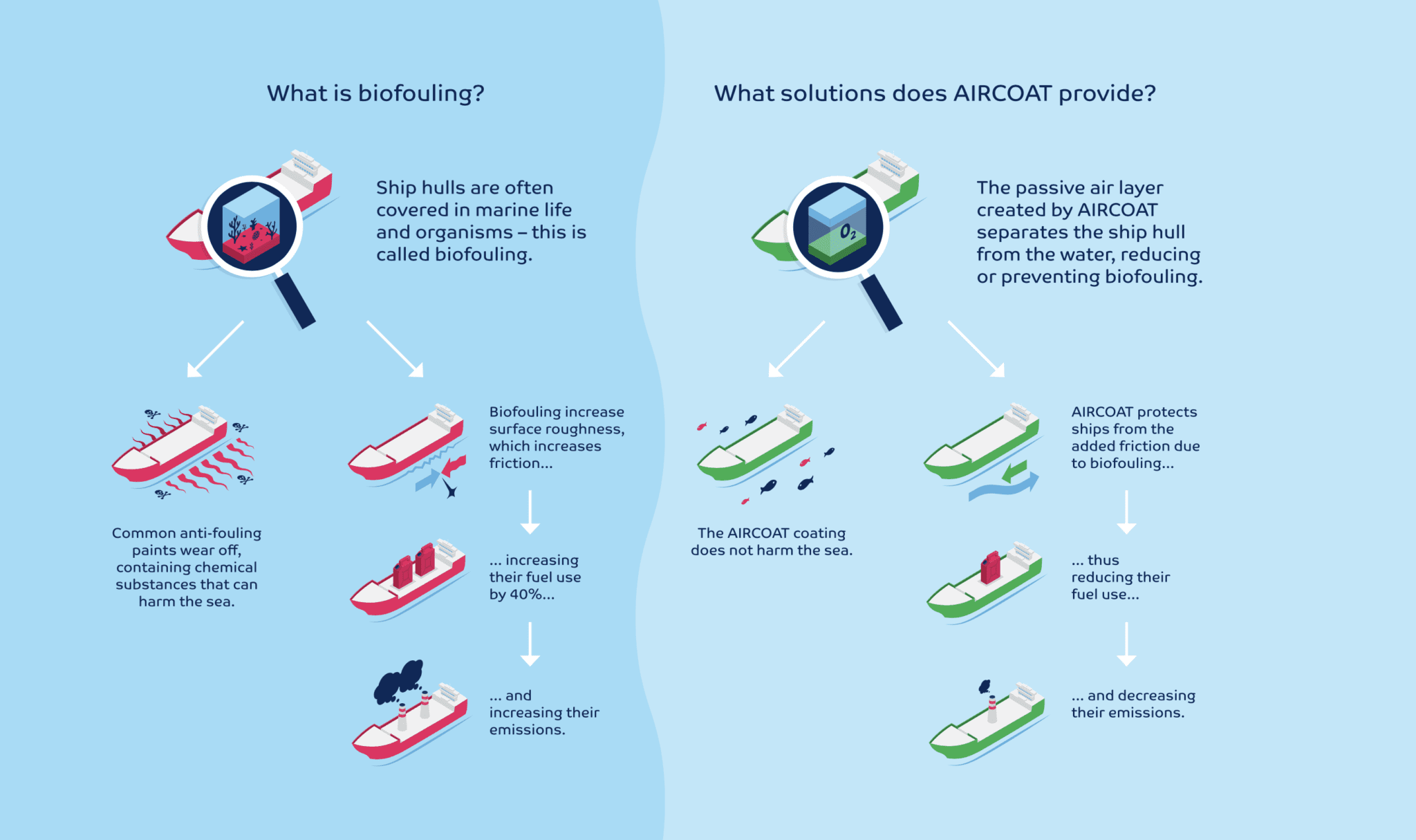
Within the project, remarkable progress was made in the development of a foil with a surface structure to retain air and to achieve drag reduction:
- Geometry and size of surface structure: theoretical calculations and simulations on high performance computer cluster have shown that drag reduction increases with surface structure and that frictional drag reduction of 10% is possible. Hence, depending on ship speed and length, the size of the surface structure has to be adapted to reach equal drag reductions.
- Production of the foil: production in a roll-to-roll process newly developed on the scale of kilometres of air-retaining foil. The individual structures on the foils are possible on the micrometre scale. This was an important big challenge to reach stability of the air layers down to higher water depths and at higher speeds.
- Application procedure of the foil on ships: industry procedures have been developed to manually apply the self-adhesive prototype foils to commercial vessel within standard dry-docking processes. Demonstration tests with a container ship showed the function applicability and operational durability of the coating.
- Test on 10-meter yacht and on a containership: in the Mediterranean Sea the yacht was coated on one side with air-retaining foil and a first demonstration on a containership in Romania under ‘real’ maritime conditions where the foil was attached to a part of the hull. The experiments are still ongoing.
- Fouling test in laboratory scale: Experiments on fouling indicate that diatoms – a special kind of microorganisms which are widespread in natural aquatic environments – avoid growing on air layers but like to grow on the same surface when the air is removed for a comparative experiment.
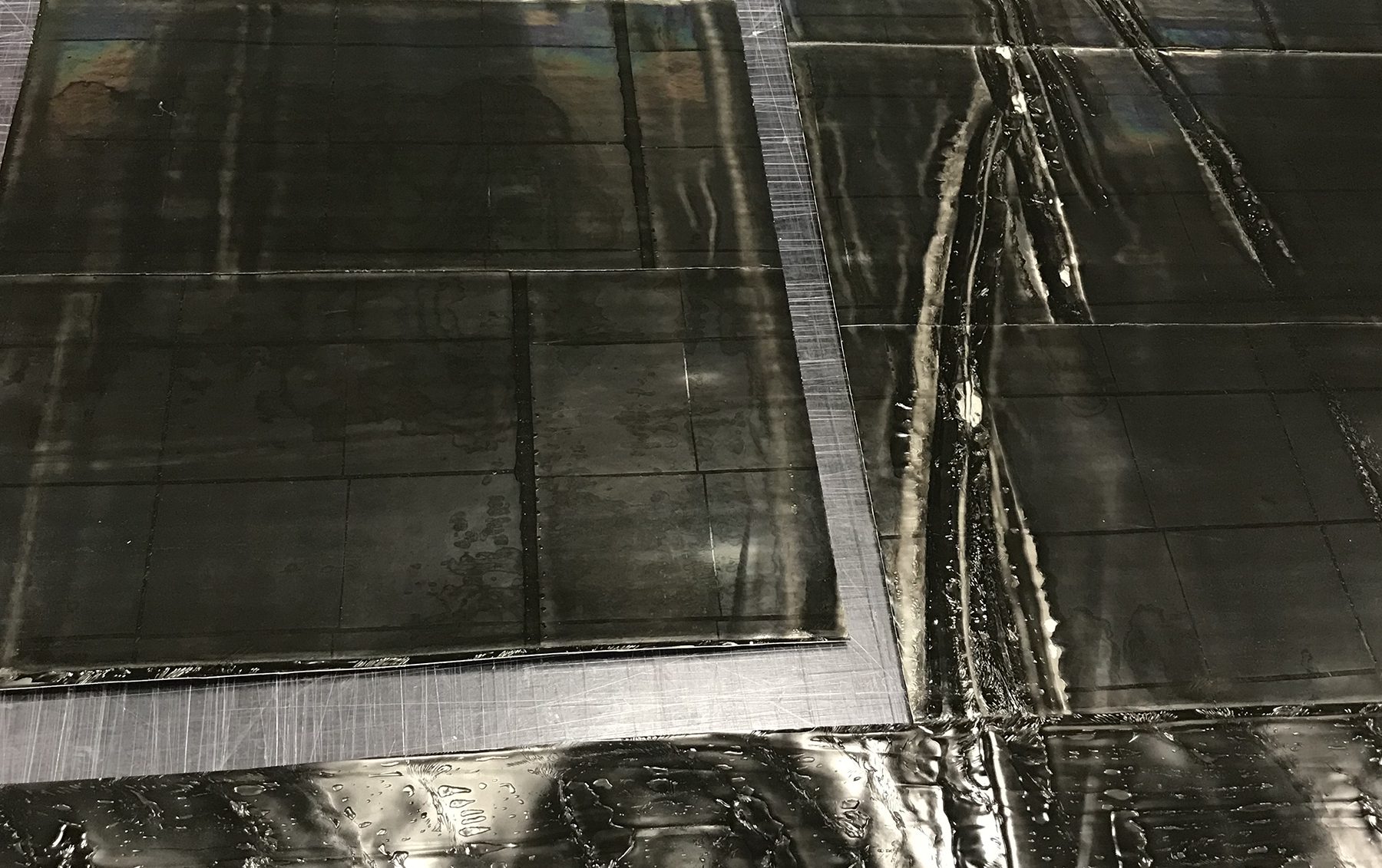
AIRCOAT foil production
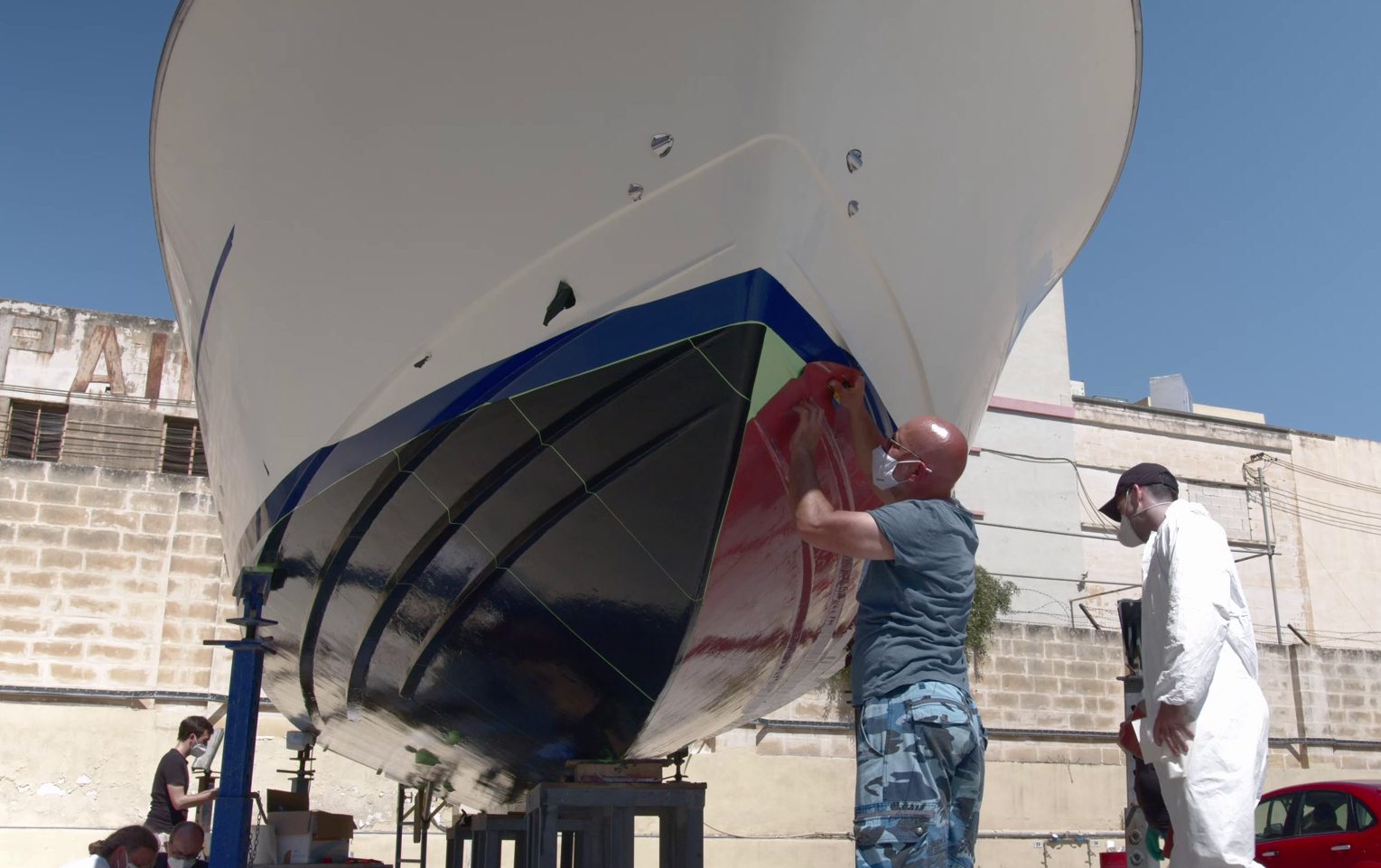
Foil application test on a research vessel in June 2021
Within the project AIRCOAT, challenges came up which have to be solved before an industrial application of the foil can be deployed:
- Degradation of air layer: three mechanisms were observed: diffusion in case of unsaturated water, mechanical stress between water and air, and hydrostatic pressure at higher water depth. The first and last mechanisms appear naturally and could be faced by refilling with air. Here, strategies are conceptualised but need to be developed. In the second case, an increase in hydrophobic surface properties and geometry of the structure seem to be promising approaches. Furthermore, hydrophilic tips of the surface structure should be considered. This is in fact the mechanism that AIRCOAT’s natural model, the Salvinia fern uses; however, the technical implementation of this on micro structure levels on large mega structures are yet to be solved.
- Long-time fouling testing in the water: Tests of the foils in the Mediterranean Sea and North Sea over several months showed growth of marine organisms on the surface of hulls. We assume that the growth was possible due to the degradation of air by diffusion. Investigations in the future with a long-time air layer have to verify this hypothesis.
- Conservation of hydrophobic property over long time periods: this is a challenge known in other fields too and has to be investigated in cooperation with specialists in surface chemistry.
- Industrial production and application procedures: although kilometres of micro-structures self-adhesive foils were already produced, in order to produce the large amount of foil needed to coat an entire large vessel. This production process needs to be upscaled which requires much larger investments in industrial equipment. Furthermore, automatic application procedures need to be developed to cut dry-dock time for market-ready future product.
This demonstrates that further developments in refilling technologies and of the materials surfaces are needed to make and keep the material more water repellent to reach better long-term stability of the air layer at higher water depths. While there was remarkable progress in structuring, fabrication and processing technologies, material issues still need improvement and more research. Furthermore, a higher number of experiments on drag reduction at relevant physical conditions would be beneficial to verify frictional drag reduction. Industrial production processes need to be set in place and application procedures automized and defined.
The combination with other hull resistance reduction measures such as fouling release, active air lubrication as riblet structures seem very promising. Given the vast market and the enormous potential to save energy with this new bio-inspired technology, it seems a good investment to work more on the remaining issues. This could make the AIRCOAT technology an important piece of the puzzle for the optimal combination of the various efficiency technologies to reach decarbonisation goals of the shipping sector. It should be noted that a complete elimination of hull friction (100% drag reduction) may lead to about 40% fuel consumption savings, not more. The 40% fuel consumption reduction represents the maximum feasible reduction limit with 100% drag reduction. The fuel used for propulsion purposes is about 75% of the total fuel consumption. The fuel needed to overcome friction is roughly half of propulsion fuel. Thus, a 10% friction reduction may lead to about 4% reduction in vessel overall fuel consumption and emissions. Further, drag reduction is dependent on vessel speed in such a way that drag reduction increases at high speeds. For slow moving cargo ships, drag reduction will be less than for fast moving vessels.
The AIRCOAT foil is introducing advanced technology in hull coatings and as such will come with strong investments later in the market from the vessel owner’s side. The owner’s CAPEX for an AIRCOAT product is anticipated to be more than any expenditures for a conventional painting. Nevertheless, what makes AIRCOAT a lucrative choice is the delta in expected operational savings. The AIRCOAT solution brings business benefits to the ship operator by materialising savings in fuel consumption and hull maintenance costs between dry dock intervals, whilst promising significant returns of investment.
CONCLUSION & RECOMMENDATIONS
It is a fascinating outlook that in the end, a technology stemming from biology – bio-inspired air-retaining surfaces – may make a strong contribution to save biological life in the sea and to preserve life on our planet. In order to achieve the climate action targets set for the transportation sector within the 2030 and 2050 horizons, the shipping sector needs to focus on a combination of quick wins that can deliver strong emissions reductions in the short and medium term. Advanced hull coatings are poised to be a key solution to improving energy efficiency, offering a potential 10% reduction of fuel consumption without the need to compromise on speed.
In the pursuit of energy efficiency gains, it is crucial that all efforts in this space also preserve the integrity of marine ecosystems, so that climate action does not have negative environmental impacts. The sector has been working to phase out harmful compounds from anti-fouling coatings since 2001, however negative impacts of anti-fouling biocides on marine life is still being detected (springeropen.com). Given anti-fouling coatings have an average duration of 5 years between applications, further incentives to the shipping industry to invest in environmentally friendly solutions to biofouling are important to ensure that solutions put in place today are contributing to both climate and marine protection goals.
In addition to offering significant fuel efficiency gains, AIRCOAT is a retrofit technology: It can be put into place on existing ships, which avoids the early retirement of the existing fleet and does not need to wait for new ships to be built. Practically, this allows for much faster adoption of changes. With 50% of the European fleet being under 15 years in age, retrofit solutions permit the industry to quickly adapt it to green solutions.
Funding cycles need to allow for real world testing when it comes to fouling as test conditions can only say so much. Funding for real world conditions requires bigger investments on a longer term into creating to scale prototypes to test.